Catalytic Advanced Oxidation
The processes of Advanced Oxidation are related to methods and reactions which use hydroxyl radicals OH. as reactive oxidative species. Hydroxyl radicals are OH groups (normally charged negatively OH-), from which one electron was stripped off. Their electric charge is therefore balanced (neutral), but their electron spin configuration is not. This imbalance makes the hydroxyl radical configuration extremely reactive towards oxidative reactions, i.e. toward capturing or sharing the missing electron from another molecule. This is how the hydroxyl radicals become the ultimate oxidation agents, capable of oxidizing other molecules.
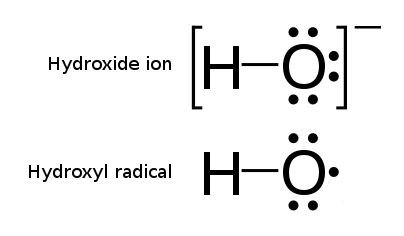
The most striking feature of the hydroxyl radicals is their oxidative potential (Eo) which is the highest of all typical oxidative agents (and second only to fluorine) which makes it the most powerful tool in oxidative reactions.
Read more: Hydroxyl Radicals Reactivity
Catalytic Advanced Oxidation is an innovative approach to ecological methods of waste water remediation and to other challenges that currently represent major unsolved pollution problems. It combines the use of the cleanest chemical - hydrogen peroxide H2O2 with Hydrogen Link's proprietary OXYCATALYST capable of incomparable generation of hydroxyl radicals.
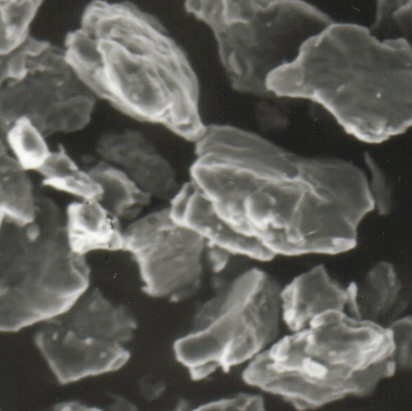
Oxycatalyst is a critical enabler for Catalytic Advanced Oxidation - a unique, proprietary heterogeneous catalyst which effectively re-routs the decomposition reaction of H2O2 into formation of hydroxyl radicals – one of the most powerful oxidative species.
Read more: Hydrogen Link's Oxycatalyst
Please contact us with inquires related to cooperation and purchase of our catalysts: Contact for Inquiry or contact@hydrogenlink.com
Oxidative reactions based on Catalytic Advanced Oxidation are the most effective way of degrading and eliminating all kinds of pollutants and waste chemicals - both in air and water. It is also one of the basic tools in a variety of chemical reactions and processes.The practical efficiency of the Advanced Oxidation relies primarily on the oxidative potential of the hydroxyl radicals and also on the abundance of the hydroxyl radicals generation in relation to the pollutant's content.
Oxidative potential of hydroxyl radicals
The "strength" of an oxidizing agent is measured by its oxidation potential - the higher the oxidation potential, the stronger the oxidative capability. This means also that the molecule of the pollutant can be only oxidized (remediated) by an oxidizing agents which have a higher oxidizing potential than the pollutant's reducing potential. Therefore, for each oxidation agent there is a range of pollutants which are chemically inaccessible for this agent due to their relative redox potentials
Species | Oxidation potential [V] |
---|---|
Fluorine | 3.03 |
Hydroxyl Radical | 2.80 |
Atomic Oxygen | 2.42 |
Ozone | 2.07 |
Hydrogen Peroxide | 1.78 |
Permanganate | 1.68 |
Chlorine Dioxide | 1,58 |
Chlorine | 1.36 |
Oxygen molecule | 1.26 |
Ref. source |
The oxidation potentials of various oxidation agents are listed in the table. The list shows a wide range of potentials for common oxidizers. Hydroxyl radical is at the top of the list, surpassing by far the atomic oxygen species, ozone and other common agents. It is clear from the table that for example ozone is not capable of oxidizing molecules that have their potential higher than 2.07 V. One such example of aromatic rings, e.g. benzene or toluene, for which Eo values in a solvent equal respectively to 2.48 and 2.26 V [ref.source]. For this reason, such aromatic rings can actually only quench ozone and they cannot undergo remediation by ozonation methods. By contrast, these aromatic rings are accessible for oxidation by the hydroxyl radical.
In practice, there are myriads of stubborn pollutants that fall into the gap between the hydroxyl radical potential and the rest of the oxidative agents, especially from the difficult pharmaceutical or chemical industries, including antibiotics, pesticides, herbicides, For such cases, Advanced Oxidation with the use of hydroxyl radicals is the only available remedy.
In general, hydroxyl radicals can induce an overwhelming variety of oxidative reactions. Examples are: either through directly adding on, for example to unsaturated or double, triple bonds, such as C=C, C=O, S=O, N=N, or hydroxylation ( introduction of hydroxyl group (-OH) into an organic compound). Alternative path involves formation of a various types of secondary radicals, according to the reaction: OH· + RH → H2O + R·
These reactions, initiated by hydroxyl radicals, proceed further depending on the effluent content and can be as complicated as the real life wastewater composition. In the case of the radical formation, the reaction can progress through more and more stable radicals (in the radical chain reaction) or through further hydroxylation using the continuous feed of the hydroxyl radicals. After the possible cascade of byproducts, finally the reaction proceeds toward thermodynamically stable oxidation products, reaching the stage of complete oxidation, so called mineralization i.e. full degradation of the pollutants.
The end products of complete oxidation (mineralization) of organic compounds are carbon dioxide (CO2) and water (H2O) only. Together with the fact that Catalytic Advanced Oxidation does not introduce by itself any other reagents or chemicals (as for example iron salts in Fenton reaction, or the electrolytes in electrochemical methods, or toxic agents as chlorine or bleach) but only hydrogen peroxide - this method has the cleanest and most environmentally friendly approach.
In reality, the effluent rarely consists of only organic compounds without the presence of elements other than C, O and H to produce only CO2 and water. In some cases (for example in oxidations of proteins, poly-peptides, amines, amides) the nitrogen groups result if the additional formation of nitrogen N2. On the other hand, oxidation of gases (CO or NO) with hydroxyl radicals results in the formation of CO2 and NO2. Depending on the initial effluent composition, the final product may consist of oxides, metallic precipitates, and sediments as well as other insoluble, oxidized compounds. The quantities of such sediments are usually very small and totally incomparable to sludge quantities produced by other methods.
The variety of oxidative reactions enabled by hydroxyl radicals makes them the ultimate oxidation tool. They can break double bonds (C=C, N=N), degrade hydrocarbons, cause epoxidation and aromatic ring opening, radical polymerization, formation of secondary radicals and many other types of reactions. Catalytic Advanced Oxidation can be very effective in decoloration and degradation of the most stubborn dyes - AZO DYES (containing double N=N bonds). It represents an environmentally friendly approach to target a multitude of pollutants and contaminants, such as in air and waste water, to remove toxic or non-degradable materials. Catalytic Advanced Oxidation deals with the removal of aromatics, pesticides, herbicides, petroleum constituents, volatile organic compounds (VOC), petroleum hydrocarbons, chlorinated hydrocarbons, dyes and organic matter. It is able to decontaminate, purify and disinfect water.
In general, the effectiveness of an Advanced Oxidation Process is proportional to its ability to generate hydroxyl radicals. Methods which use hydrogen peroxide have already an advantage of remarkable oxidative ability of H2O2 itself (which is greater than permanganate and chlorine species - see the Table above), but being able to re-rout the H2O2 decomposition reaction into formation of hydroxyl radicals jump-starts the oxidative potential to a supreme level. Therefore, a catalyst capable of such action becomes a unique enabler for a powerful oxidative technology: Catalytic Advanced Oxidation.
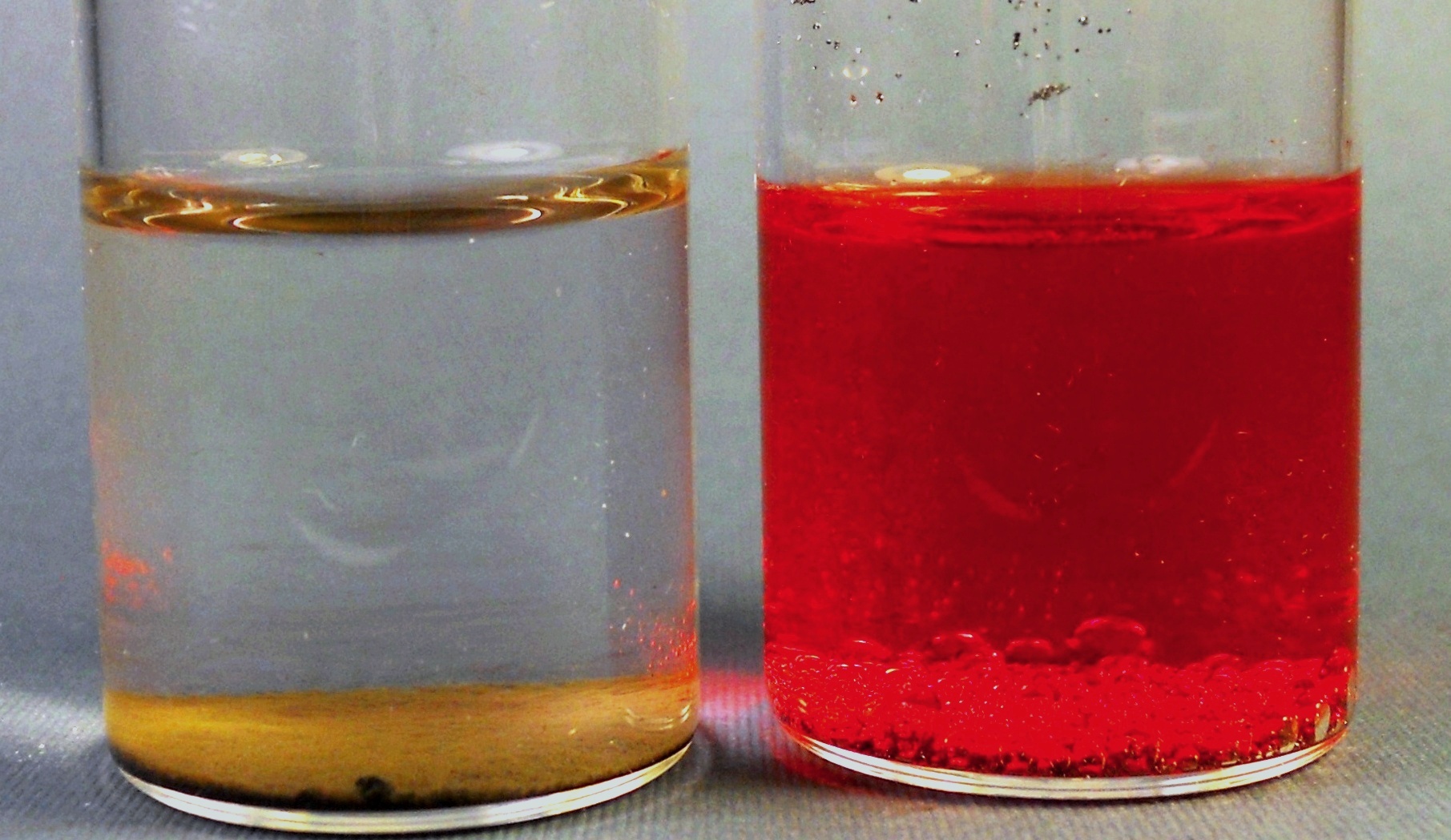
Several catalyst are known to decompose hydrogen peroxide (such as manganese dioxide, silver, platinum etc,), but this type of decomposition mechanism involves mainly generation of oxygen and only a very limited number of hydroxyl radicals. Our unique oxycatalyst, which is based on nano-technology, is actually capable of producing unparalled amounts of hydroxyl radicals, which is illustrated by the following experiment. A solution of a red-colored Azo Dye (Allura Red) was subjected to hydrogen peroxide addition with a catalyst. The Oxycatalyst was added to the left-side vial in the photograph and manganese dioxide was added to the right side vial. The hydrogen peroxide with MnO2 was decomposed with the generation of oxygen, but neither the hydrogen peroxide itself nor the formed oxygen species were able to remove the color of the dye. By contrast, the oxycatalyst was able to cause full decoloration of the red dye very quickly. The reason for such difference is that the color of the Allura Red dye is related to the presence of double bonds N=N, which only the hydroxyl radicals are able to attack and neither H2O2 nor oxygen species are capable of oxidizing them and degrading the dye.
Read more: Dye decoloration and degradation Hydroxyl radicals reactivity
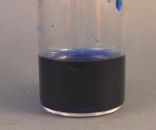
Catalytic decomposition of hydrogen peroxide can be also evaluated with Methylene Blue, which is the known indicator of hydroxyl radicals. In the presence of hydroxyl radicals Methylene Blue undergoes decoloration, even in hydrogen peroxide environment which is not a reducing agent. The decoloration of Methylene Blue is instant and fully accomplished within minutes.
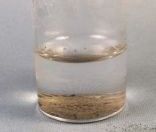
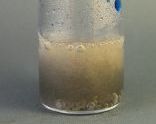
Catalytic generation of Hydroxyl Radicals
Oxycatalyst advantages - summary
- Oxycatalyst is uniquely capable of generation of an abundant amounts of highly active species - hydroxyl radicals OH· that exhibit unsurpassed oxidative capability
- no other chemicals or ingredients are needed than hydrogen peroxide H2O2 - the "cleanest chemical". Thus, no additional pollution is introduced by the treatment itself. This is in contrast to electrochemical AOP which introduce electrolytes or Fenton AOP which needs soluble iron salts, or flocculation
- Oxycatalyst is heterogeneous (i.e. in the solid state) and it is based on specific hetero-complex formed through nano-technology. Therefore it does not become part of the solution and can be recovered and reused multiple times
- Oxycatalyst enables easy adjustments of the kinetics of the reaction, depending on the requirements. There is a range of forms of the catalyst - from a fine powder to granulate of more than 5 mm, which can be used in various types of systems
- Oxycatalyst operates best at neutral pH = 7, but it can also be used in a wide range of pH - from alkaline to acidic. It does nor require pH adjustments (such as in the case of electrochemical methods and Fenton).
- Oxycatalyst is stable and controllable and does not deteriorate upon exposure to air or moisture or after prolonged storage
- Oxycatalyst can be stored indefinitely without any loss of their activity and structure, without protective atmosphere
- Oxycatalyst does not need activation or regeneration or any kind of preparation or reduction process
- Oxycatalyst has very high efficiency combined with low cost - it contains no noble or exotic elements. It contains no toxic or dangerous components
- Catalytic Advanced Oxidation does not require any external energy input and no expensive instrumentation.
- The method does not produce any sludge or unwanted byproducts, only very small amount of fully oxidized (mineralized sediment).
- Although the hydroxyl radicals are powerful and reactive, they do not survive in the application with hydrogen peroxide to become risky. The radicals are very short-lived and unstable, so they can either oxidize the chemicals in the effluent, or must instantly end up in oxygen molecules, thus becoming the main byproduct of Catalytic Advanced Oxidation, along with the water molecules.
Please contact us with inquires related to cooperation and purchase of our catalysts: Contact for Inquiry or contact@hydrogenlink.com
Catalytic advanced oxidation with solid hydrogen peroxide source
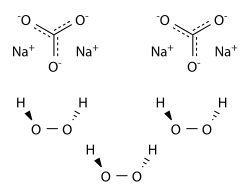
Hydrogen peroxide in Catalytic Advanced Oxidation can be alternatively replaced by solid forms of hydrogen peroxide, such as adducts of H2O2 with sodium carbonate or urea (i.e.sodium percarbonate SPC and percarbamide).
Sodium percarbonate (SPC) - Na2CO3·1.5H2O2 - is a crystalline adduct of H2O2 with sodium carbonate (“ash soda”). It is a convenient and concentrated source of hydrogen peroxide., easy to handle and transport. The amount of hydrogen peroxide by weight in sodium percarbonate exceeds 30%. It can be handled more conveniently and more safely than the same concentration of H2O2 in water solutions. Sodium percarbonate is used in many laundry detergents and cleaning products which are environmentally friendly, such as OxiClean and Vanish.
In water, sodium percarbonate releases hydrogen peroxide according to the reaction:
2Na2CO3 . 3 H2O2 → 2Na2CO3 + 3H2O2
Catalytic decomposition of hydrogen peroxide from sodium percarbonate is equally efficient as from the water-based H2O2. However, the main difference is in the resulting pH level. Sodium percarbonate introduces alkalinity in the solution, which may change the specific routes of the oxidative reactions of the pollutants in the Catalytic Advanced Oxidation. In some cases, depending on the specific chemistry of these molecules, the alkaline environment may promote the oxidation reaction, but in some cases the alkalinity may impede for example decoloration reactions of certain dyes. On the other hand, the introduction of alkalinity of the SPC into already acidic effluent may bring the pH to more neutral level and ease the remediation. Therefore advantages of the use of SPC need to be evaluated depending on the content and chemistry of the effluent.
The efficiency of the Catalytic Advanced Oxidation is related to the formation of hydroxyl radicals. The following test shows the Methylene Blue solution to indicate generation of hydroxyl to for the reaction with SPC catalyzed by the Oxycatalyst.
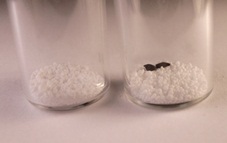
in the right vial
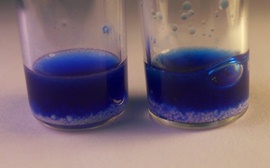
In this test, the Oxycatalyst is added to the solid powder of SPC - in the right vial only. Next, a Methylene Blue solution is added to both vials, initiating dissolution of the sodium percarbonate and release of hydrogen peroxide from the SPC compound. It can be seen that the catalyzed mixture (right vial) starts the reaction immediately, while there is not much activity without the catalyst. The solution of Methylene Blue undergoes decoloration until it is completely transparent and the overall reaction ceases in the right vial.
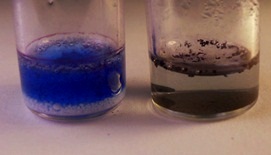
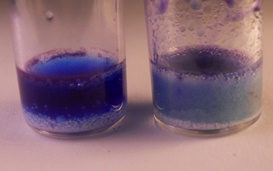
In such reactions, various kinds of pollutants can be eliminated - including organic matter, dyes and chemicals. Catalyzed oxidation is much more efficient than the action of the sodium percarbonate alone. As mentioned above, however, sodium percarbonate introduces alkalinity, which might be not advantageous for some types of compouds. for which either neutral or acidic environment is preferable.
The use of the technology of Catalytic Advanced Oxidation relates not only to homogeneous reactions (where the reagents are soluble in water solutions of H2O2) but also in a variety of heterogeneous reactions, where the oxidized substance is in another phase, e.g. gas or a hydrophobic chemical. This includes oxidation of greenhouse gases such as CO or NOx being oxidized in a catalyzed hydrogen peroxide bath.
The critical feature of the Oxycatalyst - i.e. the ability to generate one of the most powerful oxidative species – hydroxyl radicals can be directly evidenced in another simple test with Methylene Blue solution. Methylene Blue (methylthioninium chloride) is a sensitive indicator of the presence of hydroxyl radicals, because it can undergo decoloration in the oxidative environment only through the interaction with hydroxyl radicals. Otherwise, the decoloration of Methylene Blue is observed only in reduction reactions.
In this experiment, the solution of Methylene Blue was treated with hydrogen peroxide catalyzed by two different catalysts: manganese dioxide (MnO2) and the Oxycatalyst. The amounts of the hydrogen peroxide and the catalysts were identical in both cases. The picture below shows the comparison of three vials: the left vial shows the initial Methylene Blue solution with hydrogen peroxide, the middle vial contains MnO2 and the right vial contains the Oxycatalyst. The reaction in the vial with the Oxycatalyst was much faster and was finished within minutes. The reaction in the vial with manganese dioxide was much more sluggish. It took minutes for the Oxycatalyst and hours for MnO2. The color in the manganese dioxide vial did not change very much, indicating very limited (if at all) generation of hydroxyl radicals. On the other hand, the methylene blue solution in the right vial with the Oxycatalyst was already totally transparent after several minutes, without any signs of the blue color.
This simple test demonstrates the generation of the powerful hydroxyl radicals by the Oxycatalyst.
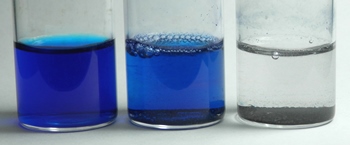
Catalytic Advanced Oxidation variations
This technology can be used in many additional variations and aspects, being applied for example in special, customized reactions.
Catalytic materials exhibit extraordinary properties when combined with a suitable environment. For example, our catalytic complexes can be efficient in the generation of radicals in other types of liquids, solvents or solutions (such as alcohols, ionic an non-ionic solutions, salts, gels, gases, vapors, electrolytes, monomers or polymers, organic liquids, or mixtures and melts) They have the ability to generate various highly reactive radicals (not only hydroxyl radicals) such as oxygen radicals, hydrogen radicals, methyl radicals, peroxide radicals etc. Thus generated radicals can be used in subsequent reactions with other substances which are susceptible to the radicals reactivity or in the respective radicals chain reactions.
The use of the technology relates not only to homogeneous reactions (where the reagents are soluble in water solutions of H2O2) but also in a variety of heterogeneous reactions, where the oxidized substance is in another phase, e.g. gas or a hydrophobic chemical. This includes oxidation of greenhouse gases such as CO or NOx being oxidized in a catalyzed hydrogen peroxide bath.
Another aspect of the process of using the catalytic complexes for the generation of radicals is related to the use of the electrostatic field or electric charge (current) in the vicinity of these materials, either within the electrolyte or in the close proximity of the electrodes. In this case, the application of electric current or the electrostatic field significantly enhances the generation of radicals and subsequently improves the performance of the system. The typical systems to improve are electrolysis systems or fuel cell systems, or photovoltaic systems.
Please contact us with inquires related to cooperation and purchase of our catalysts: Contact for Inquiry or contact@hydrogenlink.com
Read more
- Hydroxyl Radicals Reactivity
- Organic Matter Degradation
- Wastewater treatment
- Dye decoloration and degradation
- Cellulose Fibers Upgrading
- Oxygen Generation
Examples of applications:
(please click on the image to watch video demonstration)
Catalytic Advanced Oxidation of Biomass and Organic wastewater
- purification and disinfection of water at pH=7, COD/COD reduction
Biomass and Organic wastewater decontamination and purification
by Catalytic Advanced Oxidation with Oxycatalyst
Wastewater with Azo Dyes- decoloration and degradation
by Catalyzed Advanced Oxidation
Dairy wastewater treatment and purification by Catalytic Advanced Oxidation -
(COD/BOD reduction)
Cellulose bast fibers upgrading and cottonization
by Catalytic Advanced Oxidation with oxycatalyst
Degradation of Cellulose by Catalytic Advanced Oxidation - catalysed Hydrogen Peroxide
Methylene Blue decoloration evidencing the hydroxyl radicals formation
in Catalytic Advanced Oxidation
General types of Advanced Oxidation Processes - in comparison with the Catalytic Advanced Oxidation
Ozonation
In the ozonation technology, hydroxyl radicals are formed when ozone O3 is decomposed in water. Ozone is a strong oxidant, but it is much less powerful than hydroxyl radicals because of its lower oxidation potential (Hydroxyl Radicasl Reactivity). Decomposition of ozone requires high pH (>10) and ozone treatment of organic chemicals proceeds faster in alkaline solutions. Therefore, effluents with neutral or acidic pH nedds to be alkalized, thus contributing to sludge formation. The ozone treatment is relatively expensive with its high energy input and capitals cost of the equipment. The most important disadvantage of ozone is however that it is very harmful when inhaled (EPA warning)
Hydrogen Peroxide and Ozone (H2O2/O3)
When O3 and H2O2 are simultaneously added to water, they participate in a complex chain of reactions and as a result this treatment is more effective than either ozonation or hydrogen peroxide alone. However, the usage of hydrgen peroxide adds to the cost of ozonation alone.
Photocatalytic oxidation processes
Advanced Oxidation Processes can be enhanced by using ultraviolet (UV) radiation, which induces formation of free radicals. Ultraviolet irradiation can be used together with ozone (O3/UV ) or hydrogen peroxide (UV/H2O2). Degradation of organic compounds occurs by hydroxyl radicals reaction, in addition to direct photolysis and oxidation by molecular ozone or hydrogen peroxide. Generally, UV treatment with ozone is more effective than UV in combination with H2O2
Advanced Oxidation treatment using a combination of ultraviolet irradiation catalyzed with TiO2 is based on the illumination of the titanium dioxide (which is a semiconductor) with ultraviolet light. This results the excitation of its valence band electrons to the conduction band and the formation of holes. Adsorbed water molecules take part in the reaction of producing hydroxyl radicals, while superoxide anion radical (O2•) is also generated. The problems with this method are related to fouling of the TiO2 catalyst in highly contaminated slurries, which on one hand reduce UV light penetration throughout the waste stream and on the other hand can block the access of the UV to the clean surface of TiO2 (a critical step for the excitation effect). In addition, the reaction efficiency is highly pH dependent.
The major problem with all photocatalytic methods is that they are energy and cost intensive. Also, turbidity and lack of transparency for UV light in highly colored or contaminated effluents impedes the efficiency of these processes.
Fenton Reaction Fe2+ / H2O2
Fenton's reagent is a solution of hydrogen peroxide and an iron catalyst. Iron (II) sulfate is a typical iron compound in Fenton's reagent.
Hydrogen peroxide reacts with ferrous iron (II) to form ferric iron (II) complex) that subsequently reacts to form hydroxyl radicals.
Fe2+ + H2O2 → Fe3+ + OH· + OH−
Fe3+ + H2O2 → Fe2+ + OOH· + H+
In the second reaction, iron (III) is reduced back to iron(II), along with the formation of a peroxide radical and a proton, as a result of the hydrogen peroxide disproportionation.
The above reactions cycle iron between the ferrous and ferric oxidation states until the H2O2 is fully consumed, producing •OH in the process.
The Fenton process requires adjustment of the solution toward acidic, i.e. low pH (2 to 5). If the pH is too high, the iron precipitates as Fe(OH)3. This is a drawback, because the usually highly alkaline textile-processing wastewaters (with high pH) cause large volumes of waste sludge which are generated by the precipitation of ferric iron salts and the process loses effectiveness as H2O2 is catalytically decomposed to oxygen.
In comparison to ozonation, the Fenton process is relatively less expensive and results generally in a larger chemical oxygen demand (COD) reduction. COD represents the amount of oxygen that is needed by the water in the decomposition and oxidation processes of organic matter, inorganic matter or chemicals.
Fenton’s process requires less energy compared to other oxidation technologies that use O3 or UV.
Read more
- Hydroxyl Radicals Reactivity
- Organic Matter Degradation
- Wastewater treatment
- Dye decoloration and degradation
- Cellulose Fibers Upgrading
- Oxygen Generation
Please contact us with inquires related to cooperation and purchase of our catalysts: Contact for Inquiry or contact@hydrogenlink.com